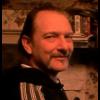
Gene Therapies and DNA Repair
#61
Posted 04 May 2004 - 12:16 AM
#62
Posted 28 May 2004 - 04:00 PM
DNA Methylation is becoming well know as a key method of gene regulation. This study shows that the researchers are beginning to seriously think about using methylation manipulation as a way of combatting age-related changes to our genes.
Public release date: 27-May-2004
Contact: Sarah Huoh
jonweine@usc.edu
323-442-2830
University of Southern California
The silence of the genes
USC researchers provide unique view of inherited disorders, cancer with discussion of new field of epigenetics in journal Nature
Los Angeles, CA, May 27, 2004-Researchers from the USC/Norris Comprehensive Cancer Center are heralding an entirely new approach to the treatment of aging, inherited diseases and cancer in a review paper published in today's issue of the journal Nature. Dispelling the belief that the only way to treat such conditions is by fixing or replacing damaged genes, they are instead focusing on the field of epigenetics--the study of changes in gene silencing that occur without changes in the genes themselves.
Many genes in our bodies are permanently turned off as part of normal development. But sometimes that process goes awry, turning off genes that should otherwise remain active. The new field of epigenetic therapy, put forth by the USC researchers in their Nature review paper, aims to switch these genes back on.
In their article, Peter Jones, Ph.D., director of USC/Norris and Distinguished Professor of Biochemistry and Molecular Biology and Urology at the Keck School of Medicine of the University of Southern California, and his colleagues lay out their new perspective on the treatment of genetic disorders by discussing the potential ways to interfere with epigenetic gene silencing, and the ways in which that potential is already being exploited.
"The fact that many human diseases, including cancer, have an epigenetic etiology has encouraged the development of a new therapeutic option that might be termed 'epigenetic therapy,'" Jones and his colleagues write. They add that a number of chemical compounds have been found that have an affect on some form of epigenetic gene change, and note that "several of these agents are currently being tested in clinical trials," including trials being conducted at USC/Norris.
This Nature review comes just days after the U.S. Food and Drug Administration (FDA) approved the epigenetic inhibitor azacitidine (Vidaza, Pharmion Corporation) for the treatment of a pre-leukemic bone-marrow disorder known as myelodysplastic syndrome, or MDS. MDS, which is characterized by the production of abnormal, immature blood cells, affects between 10,000 and 30,000 people each year, is most prevalent in people over age 60, and can be fatal. Until now, there was no approved treatment for MDS.
Azacitidine was first synthesized in the 1960s in Czechoslovakia; it received its first exposure in the United States in the Childrens Hospital Los Angeles laboratory of then-fellow Peter Jones. Although the drug had initially been envisioned as a chemotherapy agent, Jones showed that it had great utility in the laboratory because it could turn on genes that had been previously locked down by methylation--a type of epigenetic change in which a methyl group becomes physically attached to the region of a gene that regulates its production of protein, shutting it down.
But Jones, who is one of the world's pre-eminent epigenetics experts, says that azacitidine's approval is bigger than its role in MDS.
"This is the first approved drug in a new kind of therapy-epigenetic therapy," Jones notes. "That gives it tremendous potential importance not just in this disease, but in a host of others as well."
Indeed, cancer and its relatives are far from the only conditions that may be affected by epigenetic gene silencing, Jones notes. A number of other diseases--most notably several that can lead to intellectual disabilities--appear to have epigenetic roots. Among them are Fragile X syndrome, Angelman syndrome, Prader-Willi syndrome and Rett syndrome. Jones also sees the application of epigenetics to combat disorders caused by aging, providing the opportunity to turn on the genes shut down by the aging process.
And the search for the right drugs to undo the epigenetic damage is equally wide-ranging. Jones is involved in research into the utility of a compound called azadeoxycytidine--a more specific version of azacitidine that only affects DNA and thus potentially carries fewer side effects. Many of the major pharmaceutical companies have at least one methylation inhibitor trial ongoing, Jones adds, and there are dozens of additional compounds being screened for their potential utility.
"It is apparent that we are just at the beginning of understanding the substantial contributions of epigenetics to human disease, and there are probably many surprises ahead," Jones and colleagues note in their review. "Elucidating the whole bandwidth of epigenetic mechanisms is an exciting challenge and will eventually lead to a clearer understanding of the development of human disease and direct therapeutic concepts into new directions."
###
Gerda Egger, Gangning Liang, Ana Aparicio, Peter Jones, "Epigenetics in human disease and prospects for epigenetic therapy." Nature, 429:457-463, 27 May 2004.
--------------------------------------------------------------------------------
#63
Posted 11 August 2004 - 10:10 PM
Procrastinating primates can be turned into workaholics, thanks to gene therapy. The discovery, which sheds light on the workings of the brain's reward centre, may further our understanding of mood disorders, such as depression and obsessive-compulsive disorder.
Like many humans, monkeys tend to slack off when their goal is distant, then work harder as a deadline looms. But when a key gene is turned off, the primates work hard from the word go, researchers report in PNAS Online1.
"The gene knockdown triggered a remarkable transformation in the simian work ethic," says Barry Richmond of the National Institute of Mental Health in Bethesda, Maryland, who studied the animals.
#64
Posted 13 August 2004 - 11:19 AM
Edited by wolfram, 15 August 2004 - 06:42 PM.
#65
Posted 10 November 2004 - 08:33 PM
DNA methyltransferase gene dDnmt2 and longevity of Drosophila.
Lin MJ, Tang LY, Reddy MN, Shen CK.
Institute of Molecular Biology, Academia Sinica, Taipei 11529.
The DNA methylation program of the fruit fly Drosophila melanogaster is carried out by the single DNA methyltransferase gene, dDnmt2, the function of which is unknown before. We present evidence that intactness of the gene is required for maintenance of the normal life span of the fruit flies. In contrast, overexpression of dDnmt2 could extend Drosophila lifespan. The study links the Drosophila DNA methylation program with longevity/aging, and has interesting implication on the eukaryotic DNA methylation programs in general.
PMID: 15533947 [PubMed - as supplied by publisher]
Link to Abstract
#66
Posted 09 December 2004 - 08:03 PM

(FYI: Didn't come with article.)
Researchers at UT Southwestern Medical Center at Dallas have successfully tested the first gene therapy for skin cancer, using a mouse model for the disease xeroderma pigmentosum, or XP.
Their results, available online and to be published in an upcoming issue of the Proceedings of the National Academy of Sciences, show promise for similar gene therapy to be pursued in children suffering from this rare disorder.
XP is a debilitating disease in which patients must avoid the sun and all other sources of ultraviolet (UV) light. Exposure to UV light increases the risk for all cancers, but exposed skin is most prone to the disease.With a 10,000-fold increase in cancer risk, many XP sufferers eventually succumb to tumors at an early age.
Mice with mutations in the gene Xpa suffer from XP and develop cancerous lesions on their skin within three weeks after UV light exposure. Dr. Errol Friedberg, professor and chair of pathology at UT Southwestern, in collaboration with Dr. Carlos F.M. Menck of the Institutes of Biomedical Sciences in Sao Paulo, Brazil injected the normal gene into mice suffering from XP. After treatment with the normal gene, the mice were free from disease.
"Gene therapy for XP has the potential to completely prevent cancer in a group of patients who otherwise may suffer no other ill effects from their genetic defect," Dr. Friedberg said.
When the body is exposed to UV light, the DNA in dividing cells can become damaged. Normally, the body enlists a group of proteins whose job it is to repair the sites of UV-induced damage. But in children with XP, mistakes in DNA caused by UV light cannot be fixed because of mutations in the genes for the repair proteins. DNA damage goes uncorrected, and as cells divide they accumulate numerous mutations. When these mutations occur in genes that normally suppress cancer, cells develop abnormally and cancer ensues.
A mutation in any one of seven human genes involved in DNA repair is sufficient to cause XP. One of these genes is XPA. Humans with mutations in XPA are one the largest groups of XP patients.
In their gene therapy study, Dr. Friedberg and colleagues injected the normal version of mouse Xpa into the mutant mice, using a disabled virus that infects multiple cells. They then exposed the mice to UV light for a few hours over several days. Five months after the last exposure – long after Xpa mutant mice would normally develop skin lesions – the treated mice merely had sunburn.
The skin cells surrounding the site of the injection in the treated Xpa mutant mice were nearly identical to those of normal animals, indicating that the DNA repair mechanism had been restored by the addition of the normal Xpa gene, Dr. Friedberg said.
Dr. Friedberg said he believes that with some technical refinement, this gene therapy technique may soon be applicable to all the mutations that cause XP in humans.
"XP is a disease that lends itself well to gene therapy, for a variety of reasons," Dr. Friedberg said. "Most importantly, skin cells are highly accessible for introducing foreign genes. Also, infection of the skin with a virus carrying the gene of interest, as we did with the mice, allows for many, many cells to receive the appropriate gene. Once some of the existing technical limitations are solved these studies can hopefully be extended to trials with human XP patients."
Link
#67
Posted 09 December 2004 - 08:13 PM
Edited by wolfram, 09 December 2004 - 08:58 PM.
#68
Posted 09 December 2004 - 10:29 PM
It's one thing to insert a specific gene into an organism's cells, but DNA damage represents loss of information, so I don't see how you can repair a gene without having some good copy of that information onhand to repair with. Repair enzymes aren't specific to repairing certain genes, they're supposed to repair any damage, as far as I can see. And even then, they are typically repairing a single-stranded defect using the complementary, and I can't understand how they know which of the 2 strands is the defect and which is the model.
Even if targetted gene repair could be achieved for a single cell, I'd worry that if applied across the whole body, it might end up accidentally "fixing something that ain't broke" (ie. misidentifying a valid DNA sequence as defective, and then modifying it)
This recombinant gene insertion experiment shown above sounds like the others before it, including the French one where the kid came down with leukemia because the gene insertion disrupted some DNA of consequence.
How can you guide/insert genes to places where they won't disrupt anything, and do this reliably across the entire human body? Gene insertion markers? If you do this, can you insert more than one gene at a time? For example, can you insert a sequence of 50 genes at once?
Because of all the subtleties of epigenetic regulation, it would seem necessary that these also be fully mapped out before therapeutic gene modification can proceed with confidence.
I would hate to get the right genes inserted inside me, only to find out that they won't be regulated properly.
#69
Posted 09 December 2004 - 11:04 PM
Back to XP, however, this is very exciting news because the next step should be to observe the effect of XP overexpression.
#70
Posted 10 December 2004 - 09:21 PM
#71
Posted 16 December 2004 - 10:16 PM
DNA Repair - common motif amongst longevity genes
http://www.imminst.o...4&t=3954&hl=&s=
and
DNA Repair - the shortest route to longevity
http://www.imminst.o...1&t=3934&hl=&s=
#72
Posted 28 December 2004 - 03:08 AM
Link: Nanoparticles pop genes into cells
--------------------------------------------------------------------------------
Ultitalented Nanoparticles Pop Genes into Cells
Can deliver drugs and DNA and allow real-time monitoring of treatment effects
Betterhumans Staff
12/27/2004 4:03 PM
Credit: Indrajit Roy et al/Proceedings of the National Academy of Sciences
Delivery vehicles: A versatile type of nanoparticle can effectively deliver genes into cells, promising a new nonviral vector for gene therapy
A versatile type of nanoparticle has shown promise for gene therapy with the ability to take up DNA, deliver it into cells and allow real-time monitoring of treatment effects.
Paras Prasad and colleagues from the State University of New York at Buffalo tested the approach using organically modified silica (ORMOSIL) nanoparticles. By tagging the nanoparticles with fluorescent dyes, the researchers could track them with a microscope.
They found that the nanoparticles could take up DNA and prevent it from degradation. To test if the nanoparticles could deliver as well as pick up, the researchers loaded them with the gene for green fluorescent protein and incubated them with cultured cells. They found that the cells fluoresced green, showing that the particles were able to deliver the gene into the cell nucleus.
The study "shows that the nanomedicine approach using ORMOSIL nanoparticles provides a promising direction for nonviral gene delivery," the researchers say. "We also established optical tracking and monitoring as useful tools for studying drug delivery and drug-cell interactions."
No viruses required
Nonviral vectors for gene therapy are thought to be safer than viruses that, while good at injecting DNA into cells, can provoke immune responses, making them less efficient and possibly harmful.
Extremely fine silica nanoparticles have attracted attention for use as nonviral vectors as they have been shown to bind and protect plasmid DNA from enzymatic digestion as well as to transfect cultured cells.
Prasad and colleagues say that ORMOSIL nanoparticles are even better than unmodified silica nanoparticles. They say, for example, that the nanoparticles can be loaded with more types of drugs and dyes and don't require the use of complex purification steps.
The researchers say that their nanoparticle approach combined with an appropriate visualization system could effectively deliver genes and drugs into cells while providing real-time monitoring of drug action.
They also say that they have successfully used ORMOSIL nanoparticles in preliminary studies to deliver genes into brain cells in living mice. This research has yet to be published.
The research using ORMOSIL nanoparticles in cell cultures is reported in the Proceedings of the National Academy of Sciences (read abstract).
#73
Posted 05 January 2005 - 08:05 AM
Attached Files
#74
Posted 10 January 2005 - 05:15 PM
CR-DNA-repair.
When I search for articles I have a long list with age-research key-searchword. This list I use to search all the sources, pubmed, altavista etc. When I find a new interesting keyword I add it to the list... ..just a tip for you articleminers.
#75
Posted 11 January 2005 - 11:01 AM

So, Wolfram, do you think increasing DNA repair function would mimic the anti-aging effects of CR?
#76
Posted 11 January 2005 - 07:08 PM
Yes I think that increasing DNA repair function could very possibly have downslowing effects on aging. Everything I have read so for in this area point in the direction that it could very well be so. But then human PARP-1 ofcourse must be able to interact with all the other mouse proteins and it is not sure that it is able to do that.
#77
Posted 12 January 2005 - 04:08 AM
CR is a diffrent story, because a whole lot of things happen during CR. Perhaps a decent case can be made that in times of reduced food availability, increased individual life span is genetically advantageous. Then it would be conceivable that CR is a pathway that evolved to affect multiple limiting factors, and thus has a substantial impact on life span.
#78
Posted 12 January 2005 - 01:14 PM
Accumulating DNA damage may be only one factor among others that cause aging, or limit viability over time.
Considering that it is the only cause of cancer (with the exception of oncogenic viruses) and that the cellular manifestations of aging, senescence and apoptosis, are the body's adaptation against cancer, it would not be an exaggeration to suggest that it is likely the most important factor.
If we slow it down, the other limiting factors may remain sufficient to maintain all, or most symtoms of aging.
If only it were that easy (to slow DNA damage down). Aside from telomerase starved chromosomes what do you think are the other limiting factors?
CR is a diffrent story, because a whole lot of things happen during CR.
Perhaps, perhaps not. The present model suggests that the cell is responding to glucose, insulin and other endocrine factors that are sensitive to glucose levels with the ultimate downstream effect being the modulation of genomic integrity maintenance mechanisms including DNA repair.
Perhaps a decent case can be made that in times of reduced food availability, increased individual life span is genetically advantageous. Then it would be conceivable that CR is a pathway that evolved to affect multiple limiting factors, and thus has a substantial impact on life span.
If indeed the case is that when an organism senses a reduction of nutrients it is able to slow down aging by modulating genomic repair mechanisms, it suggests a variable rate of aging that is coupled to the environment. More importantly, it suggests a defined, yet mutable rate of aging.
Consider an adaptation where an organism accelerates in aging rate when it is deprived of food. Why do we not see this?
#79
Posted 12 January 2005 - 02:37 PM
Consider an adaptation where an organism accelerates in aging rate when it is deprived of food. Why do we not see this?
Because evolution selected for surviving *famine*, which was arguably the most common condition encountered on some repeated basis from the savanna to the tundra, whether driven by drought, seasons, or climatic disruption and desertification. It may even have variants in marine life.
Feast AND famine were the norm for hundreds of millions (if not billions) of years that we are trying to even out. I suspect out there in the animal kingdom exists a mayfly phenomenon where all the gametic energy is packed into a single gorging moment but I do not believe even mayflies can be bothered eating as they are too preoccupied with procreating. That was the goal of lengthy larval phase before.
One other reason why CR might actually not slow metabolism down while putting us in this *survival* mode is so as to preserve strength for hunting/gathering because if an organism becomes too weak to find food it will inevitably die.
#80
Posted 14 January 2005 - 06:38 AM
Agreed.[DNA damage rate] is likely the most important factor.
Indeed.If only it were that easy (to slow DNA damage down).
Mechanistically distinct factors may includewhat do you think are the other limiting factors?
- Intracellular junk
- Extracellular junk
- Hormone level changes (inducing senescence, among other things)
- Developmental downregulation (adult stem cell depletion, immune aging, impaired signalling)
- Decreased proteasome function
- (The treatment of mitochondrial DNA damage rate should be mechanistically quite different from nuclear treatment, and easier)
For a start, the beneficial effect of CR on intracellular and extracellular junk accumulation is well documented.[a whole lot of things happen during CR.] - Perhaps, perhaps not.
I believe, the late onset of increased DNA damage repair should be much less beneficial than early onset treatment, because a large number of pre-cancerous cells are already present at advanced age that have the capacity to do microevolution against any such treatment.
#81
Posted 14 January 2005 - 11:14 AM
Nuklear DNA damage ---> Intracellular junk
Nuklear DNA damage --->Hormone level changes
Nuklear DNA damage --->Developmental downregulation
Nuklear DNA damage --->Decreased proteasome function
Nuklear DNA damage--->Defective mito function more ROS.
This doesn´t mean that that forexample intracellular junk wouln´t accumulate in the long term anyway but at least DNA-damage speeds up the process.
There is no way I can think of that enables us to make DNA-repair perfect.
I suggest increased expression of proteins participating in apoptotic pathway that would make cells more sensitive to DNA damage and defective cells would thus kill themselves more frequently. To be able to do this we must also be able to increase telomerase expression so that the remaining vital cells could divide without getting to short telos.
#82
Posted 14 January 2005 - 08:21 PM
#83
Posted 14 January 2005 - 11:03 PM
I believe, the late onset of increased DNA damage repair should be much less beneficial than early onset treatment, because a large number of pre-cancerous cells are already present at advanced age that have the capacity to do microevolution against any such treatment.
By then it is possible that the severity of DNA damage has forced the cell to alter its gene expression patterns so that it is routed into one of three fates: tumorgenicity, apoptosis or senescence. The last two are protective countermeasures to mitigate against the first. The question then becomes: can the cell be rescued or is it transcriptionally locked? For how long after damage has occurred can DNA repair mechanisms still be effective? This depends on the mechanism and falls is the realm of further experiment. Undoubtedly DNA repair should be effected as soon as possible after the lesion has occured. However we know that a cell has tremendous genetic redundancy, particularly specialized cells that only require a limited portion of their genome to be functional. We also know that transcriptional behavior can altered.
Aside from cerebral neuron cells whose axonal and dendritic spatial arrangement is necessary to maintain host memory and identity, all other cells can be allowed to die and replaced from a local stem cell pool including glial cells. This consideration decreases the magnitude of the problem to one cell type in one tissue compartment.
Mechanistically distinct factors may include
- Intracellular junk
- Extracellular junk
- Hormone level changes (inducing senescence, among other things)
- Developmental downregulation (adult stem cell depletion, immune aging, impaired signalling)
- Decreased proteasome function
- (The treatment of mitochondrial DNA damage rate should be mechanistically quite different from nuclear treatment, and easier)
Any type of altered transcriptional activity can contribute to the above so minimizing DNA damage would still have a positive effect.
In order to better understand this, imagine the transcriptional behavior and phenotype of a hypothetical cell that can never have its genomic or mitochondrial DNA damaged.
#84
Posted 17 January 2005 - 04:19 PM
#85
Posted 17 January 2005 - 11:23 PM
What did you have in mind?
#86
Posted 19 January 2005 - 06:33 AM
DNA damage can be detected by cellular machinery, but mutations after they happened as a result of damage cannot. Once they are there, mutations therefore cannot activate the apoptotic pathway and some, as you said, will always slip past our controls.I suggest increased expression of proteins participating in apoptotic pathway that would make cells more sensitive to DNA damage and defective cells would thus kill themselves more frequently. To be able to do this we must also be able to increase telomerase expression so that the remaining vital cells could divide without getting to short telos.
As much as we may be able to increase DNA repair, we will only slow the rate at which damage occurs. We can reverse damage only by cellular replacement. Thus, if we want to live indefinitely, DNA repair alone in the neurons will be insufficient.Aside from cerebral neuron cells whose axonal and dendritic spatial arrangement is necessary to maintain host memory and identity, all other cells can be allowed to die and replaced from a local stem cell pool including glial cells. This consideration decreases the magnitude of the problem to one cell type in one tissue compartment.
To reverse genetic damage without cellular replacement, one would have to take science fiction to the next level, e.g. go for gene-by-gene recombination repair from ex vivo sources, or why not just uploading... I have my doubts whether we can live to see these times even with boosted neural DNA repair. Perhaps with appropriate glial rejuvenation it's possible, but I would prefer not to have to bet my life on it.
On the other hand, if neuronal replacement were performed slowly, so that new cells get time to integrate with the existing structures before you let the old ones die off, there is a chance that memory and other critical structures may be preserved. This could be proof of principled in mice. Use classical conditioning to confer some pseudo-personality trait to them, perform gradual neuronal replacement and see how the trait persists in comparison to control.
Basically that every cell, and the body as a whole throws a barrage of defenses at any such thing. Viruses cannot get through the cell membrane by brute force, but have to hijack a specific cellular surface function to do the job for them. The distribution of such surface functionality varies heavily with cell types and a single virus could not possibly serve all, or a sufficient selection, of them.What are the problems of making a virus that would infect every cell in the body?
Then comes the nuclear envelope barrier. Either get in by chance, when the envelope is being reassembled after division, which is inefficient, or find a way to trick the pore into taking you up, which inflicts a size limit of strictly 25 nm diameter.
The next major problem is the penetration of epithelia and endothelia such as the blood brain barrier. If you apply a large dose of virus, some cells behind such barriers may get no virus at all, while more exposed ones, such as near the capillary walls, will receive multiple infections.
The next problem is to get the genes inside, in a way that is permanent, even when the cells divide, and non-pathogenic to the host (e.g. integration)
I would not say that these and other problems are completely beyond solutions, but the final, and in my opinion largest problem is that very few present day gene therapy developers have an imperative to make a virus that infects every cell. Most of the conditions they (and their grant-givers) seek to cure affect only a very limited fraction of cells and they actually have considerable problems to keep their vectors out of the cells the don't want to infect.
Edited by John Schloendorn, 19 January 2005 - 06:57 AM.
#87
Posted 19 January 2005 - 01:58 PM
As much as we may be able to increase DNA repair, we will only slow the rate at which damage occurs. We can reverse damage only by cellular replacement. Thus, if we want to live indefinitely, DNA repair alone in the neurons will be insufficient.
1. Theoretically DNA damage could be slowed down substantially - see deinococcus radiodurans
2. Indeed increased stem cell proliferation would have to be part of the solution.
3. We can reverse damage using gene therapy where loss of function mutations can be replace with functioning genes.
4. If the damage happens to include a regulatory non-coding site then a construct can be designed that incorporates a promoter able to replace or approximate the regulatory mechanism
#88
Posted 19 January 2005 - 03:29 PM
Well radiodurens has extreem capabilities to repair chromosome brakes this doesn´t meen it has to have extrem point mutation repair. Does it? Its not hard to find out if radiodurens proteins could be used in eucharya. Super radio resistant yeast would be a good begining.
#89
Posted 19 January 2005 - 10:27 PM
1) While bacteria tell us presumably little about the aging phenotype of multicellulars, there exist even some vertebrates that seem to have found a balance between damage repair and cellular regeneration that leads to a linear aging curve, strongly arguing that it is possible

Apart from that, much of Deinococcus break repair relies on a mechanical structure that allows spontaneous reassociation of the fragments, as well as genetic redundancy. One could try to induce redundancy in the neurons by fusion with targeted stem cells, but that again may have side effects.
2) We should not throw away our unique ability to handpick and expand the best cells ex vivo, although it seems to be possible without it.
3) I called that sci-fi, because you would have to do it for every important gene in every cell. But occasionally sci-fi becomes reality...
4) Which means even some orders of magnitude more recombination vectors to be synthesized. Perhaps that's ok with tech to be developed within our life time, perhaps not.
#90
Posted 20 January 2005 - 01:26 AM
Attached Files
0 user(s) are reading this topic
0 members, 0 guests, 0 anonymous users